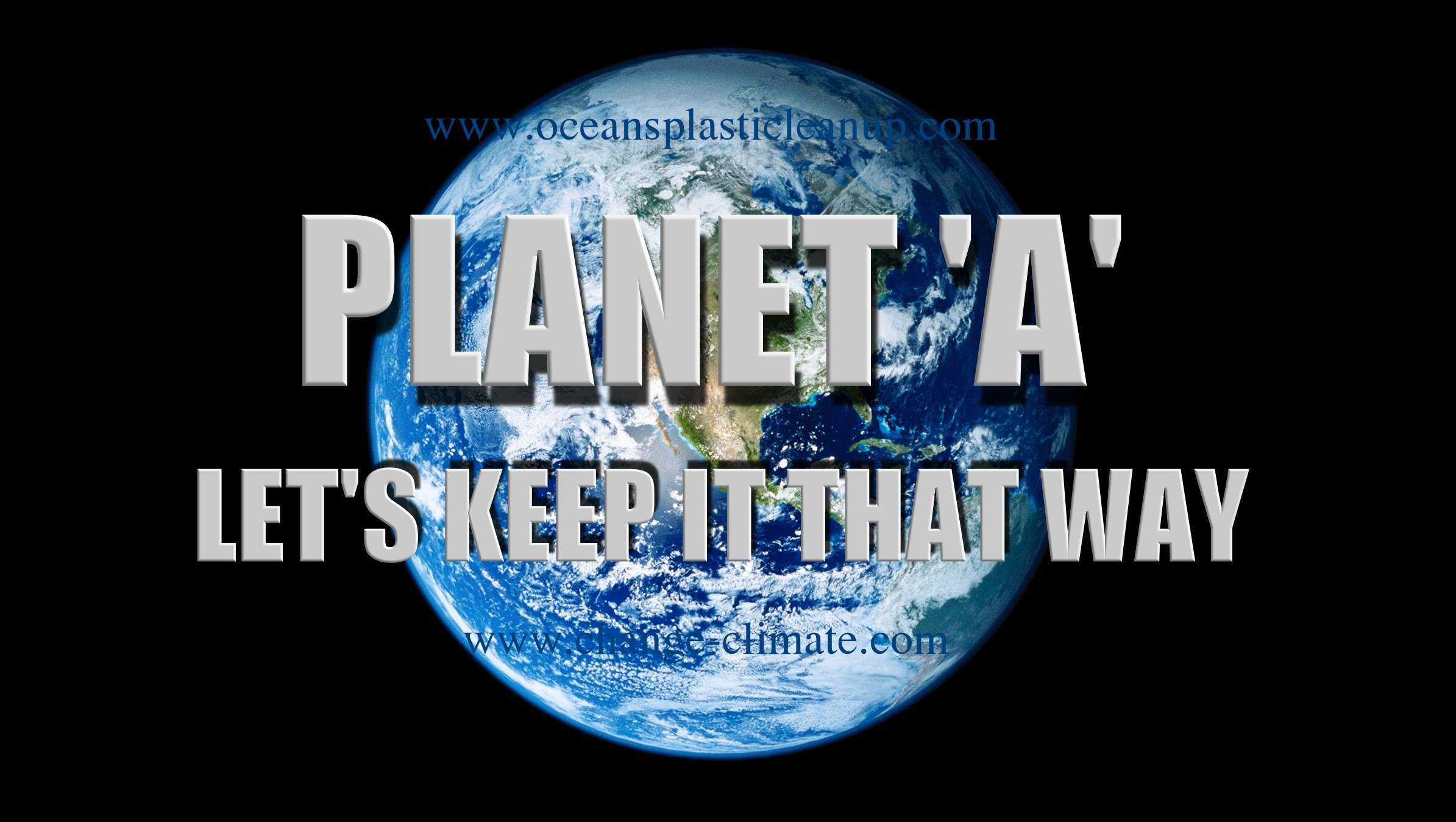
POWER -
Although some countries act as though there is no energy crisis, in fact
they have departments for such matters, that are there to advise for how
much longer they can abuse their positions in world politics, to take
advantage of other countries and keep on killing species and warming the
planet - before their kleptocratic
inclinations are compromised. The disposal of nuclear waste is an
international problem, that the popular media does not seem to have a
handle on, or is otherwise being coerced into remaining silent. We are
all being subjected to mild radiation
poisoning, that can mean triggering cancer in later life.
Atomic
fission from nuclear power
creates radioactive waste that can never be safe for humans on planet earth
because of uncertainty over long term decommissioning and management
plans. Electricity
generated using atomic fission is planned to be used by France and Russia
who want to make
pink
hydrogen, despite it being more expensive than renewables.
Does
that make sense?
No.
Why? Because the money spent installing nuclear power plants that are
dangerous, could just as easily be spent building solar
or wind harvesting plants,
where there is no pollution danger - or long term radiation contamination
issues. Why
then would Emmanuel
Macron want to back EDF
or Rosatom, in building toxic waste dumps on their doorstep. The only answer
we can think of is short term economics. The French president has sold his
environmental credentials to the highest bidder, and in the process, given
Russia another foothold in Europe, along with the Gazprom natural gas
fiasco. And look what they are doing there, holding Europe to ransom. But
get this, this unholy alliance is trying to sell the package to French
voters and the European
Parliament as 'green hydrogen.' Talk
about brazen greenwashing. Naturally,
Germany is opposing the term as misleading advertising to investors, and of
course the voting public. As with Brexit, it has been shown that voters tend
to believe whatever bullshit is being
served up by a good public speaker. Voters are not that clued up, and have
to rely on what they are hearing on television, online or in newspapers - as
being true. When mostly, especially come election time, it is very far from
the truth.
COST OF DECOMMISSIONING A NUCLEAR POWER PLANT UNCERTAINTY
- 2011
Decommissioning a Nuclear Plant Can Cost $1 Billion and Take Decades.
Spent fuel also creates new stockpiles of radioactive waste in need of disposal, with few options available.
When the Zion Nuclear Power Station in Illinois closed its doors in 1998, plant owner Commonwealth Edison, now part of Exelon, thought it would take more than two decades to clean up the site.
At the time, Zion needed repairs that exceeded the value of the 2,080-megawatt plant, and dismantling it was the better financial option, said Craig Nesbit, vice president of communications at Exelon. But when operations ceased, the flow of money from utility ratepayers also stopped, drying up the source of Zion’s decommissioning funds.
[Oh shit! Why was there no secure deposit by way of a
bond for such eventual & essential management]
The company opted to delay its cleanup plan. Aside from removing the main reactor components, Exelon would not begin the rest of the work until the 2020s, by which time the funds would have accumulated enough interest to cover the full decommissioning process.
Luckily for Exelon, though, another option presented itself when EnergySolutions, a nuclear waste managment company based in Salt Lake City, offered to take over the decommissioning plan. The company acquired the Zion plant in September 2010. According to EnergySolutions CEO Val Christensen, full decommissioning will cost about $1 billion dollars over the next 10 years.
EnergySolutions can expedite the cleanup because of its technical capacity, said Christensen. His company is currently decommissioning 18 reactors in England. They also own a low-level nuclear waste storage facility in Clive, Utah, which will speed up the waste disposal process.
The move has saved Exelon considerable headache and illuminates some of the unseen challenges of nuclear energy operation. Indeed, other plant operators haven’t been as lucky when it came to decontaminating their nuclear reactor sites.
Although the usual critiques of nuclear generation revolve around safety risks and high construction fees, relatively little attention has been paid to what happens when a nuclear plant powers down for good.
Costs Can Reach Over $1 Billion at 2011 Prices
Every nuclear plant must be decommissioned at the end of its useful life, usually after it has been operating for 40-60 years. The costly, labor-intensive process involves two major actions: nuclear waste disposal and decontamination to reduce residual radioactivity.
The U.S. currently [@2011] operates 104 commercial nuclear power plants. Most were built in the 1970s and are slated for decommissioning during the next three decades. As of April 2011, there were 23 nuclear units in various stages of decommissioning. Ten out of the 23 have been completely cleaned up.
According to Paul Genoa, director of policy development of the Nuclear Energy Institute, a trade group for the nuclear power industry, decommissioning costs typically run at $500 million per unit. But actual costs vary based on the plant’s size and design, and some have reached over $1 billion — between 10 percent and 25 percent of the cost of constructing a nuclear reactor today.
Projects can run over budget if the plant is more contaminated than previously thought, said David Lochbaum, director of the Nuclear Safety Project at the Union of Concerned Scientists.
Industry Learns from Mistakes
A decommissioned plant creates several different streams of waste. For one, spent nuclear fuel rods are kept in dry storage at the reactor sites. According to the Nuclear Energy Institute, an average nuclear plant generates 20 metric tons of used nuclear fuel annually, or 1,200 metric tons over a plant’s 60-year lifespan — an amount equivalent to the size and weight of about 1,200 SUVs.
Two, anything contaminated with small levels of radiation — pipes, tools, workers’ clothing — are sent to special low-level nuclear waste facilities around the country. The remaining non-radiated waste can be disposed of in regular landfills.
Human error can complicate the cleanup process.
When Connecticut’s Haddam Neck plant was dismantled in 1996, for instance, some contaminated materials were mistakenly sent to municipal landfills. They were later dug up and moved to nuclear waste facilities, a mistake that cost the company millions. The original decommissioning cost was estimated at $719 million; the company spent nearly $1.2 billion in the end.
“We’ve learned from those lessons,” said Genoa of the Nuclear Energy Institute. Early plants like Haddam Neck were built in the 1960s “at a time when radiation detection monitoring is worse than it is today.” The Nuclear Regulatory Commission has since strengthened safety regulations. Today’s plants, for instance, closely monitor radiation levels in the building and surrounding land to ensure that contaminated materials are disposed of in the proper facilities.
Lochbaum agreed that there is “better tracking today.” He also predicted that the process would improve with time, as the industry gains more experience decommissioning plants.
SAFSTOR’S Safety Advantages
The Nuclear Regulatory Commission offers three pathways to decommissioning. The first involves immediate cleanup after the plant shuts down. Genoa said this is the fastest method to return the site back to the public for beneficial use.
To date, all 10 of the fully decommissioned plants have met the NRC requirements for unrestricted use, which means the sites are safe enough to be reclaimed for any purpose including agriculture, housing or green space.
In the second option, called SAFSTOR, the plant is closed and awaits cleanup at a later time, offering plants like Zion extra time to increase their decommissioning funds. The NRC gives utilities up to 60 years to complete decommissioning.
This waiting period adds flexibility for plant owners with multiple reactors. The Illinois Dresden plant in Morris, Ill., for example, has three units. The first was shut down in the late 1970s, but units 2 and 3 are still running. Once they cease operation all three units will be decommissioned together to save money and resources.
Because radioactivity decays over time, SAFSTOR has some safety advantages as well, explained Genoa. Isotopes with short half-lives, like cobalt-60 (5 years), will be easier to clean up if the plant remains in stasis for decades.
Of the 13 reactors currently being decommissioned, six chose immediate decontamination and seven remain in SAFSTOR conditions.
The ENTOMB Option
No U.S. plant has ever chosen the third decommissioning option, called ENTOMB. Genoa said the name brings up unfortunate images of Chernobyl, but the comparison is not a fair one.
“In Chernobyl, the radiation was uncontained, so they built an enclosure over it to protect the public,” he said. By contrast, the ENTOMB option is much more controlled.
Workers would begin by moving the fuel rods into dry storage casks. That first step removes 99.9 percent of the plant’s radiation. Next, they use solvents and filters to clean up other contaminated surfaces. The radioactive material is sent to low-level waste facilities, and the plant is left to sit for decades.
Genoa said that with ENTOMB there is no need to build extra containment buildings because most of the radiation is already gone, though plant operators would continue to monitor the site for security. “It might need some waterproofing or coats of paint, but other than water or [unauthorized] people getting in, there’s very little risk.”
After 80 to 100 years, the plant would be safe enough to enter while wearing street clothes, said Genoa, and workers could dismantle the plant with just “a plasma torch and dust mask.”
But despite the safety advantages, Genoa said utilities don’t like the ENTOMB option because they don’t want to deal with the long-term liability. And most nuclear plants are built on valuable waterfront property, so the public generally wants to reclaim the sites as soon as possible.
Zion First to Transfer License
Zion’s decommissioning has drawn its fair share of attention. While nuclear operators often hire contractors for the cleanup work, Exelon is the first company to transfer its nuclear operating license for the purposes of decommissioning.
Christensen, the CEO of EnergySolutions, said they acquired the license to speed up the cleanup process. “By owning the site ... we can manage our own destiny, keep on schedule and make changes the split second they need to be made.
“It is also a profitable venture for EnergySolutions,” Christensen added. For example, as operators of the Clive nuclear waste facility in Clive, Utah, EnergySolutions will make money from the disposal fees.
About 30 percent of the cost of decommissioning goes towards waste disposal, said Christensen.
EnergySolutions now has total stewardship over the project. They’re also legally responsible for anything that might go wrong, but Christensen was confident in his company’s abilities.
Trading Radioactivity for Volume
Low-level radiation waste comes in three varieties: Class A, B and C. The facility at Clive only takes Class A waste, which contains the lowest levels of radiation.
In addition to Clive, there are two other low-level nuclear waste facilities in the United States — in Barnwell, South Carolina and Hanford, Washington. Both sites accept Class B and C waste but only from select northwestern and eastern states. Similarly, a new low-level waste facility under construction in Andrews County, Texas will limit its intake to nuclear waste from Texas and Vermont.
When Barnwell and Hanford started restricting their operations, nuclear plants learned to adjust their practices, said Genoa. Operators began changing filters more often to selectively create Class A waste that could be sent to Clive. As a result, Class B and C now make up less than 15 percent of low-level nuclear waste.
In decommissioning Zion, EnergySolutions will store its Class B and C waste on-site and rely on the Clive facility for disposal of Class A material, while the industry watches the Zion plant’s experience.
For his part, Genoa is supportive of the company’s unique decommissioning approach, calling the license transfer at Zion an “interesting” model for the future. The NRC will be keeping close tabs on the project, he said, in hopes that “the new approach can lead to a cheaper, more efficient [decommissioning] process.”
A
fantastic sight that happens all around the world every morning, hydrogen
fusion is happening in space to give us infinite energy. Sunrise
heralds the beginning of each new day. We can count our lucky stars that we
have this one to power life on earth.
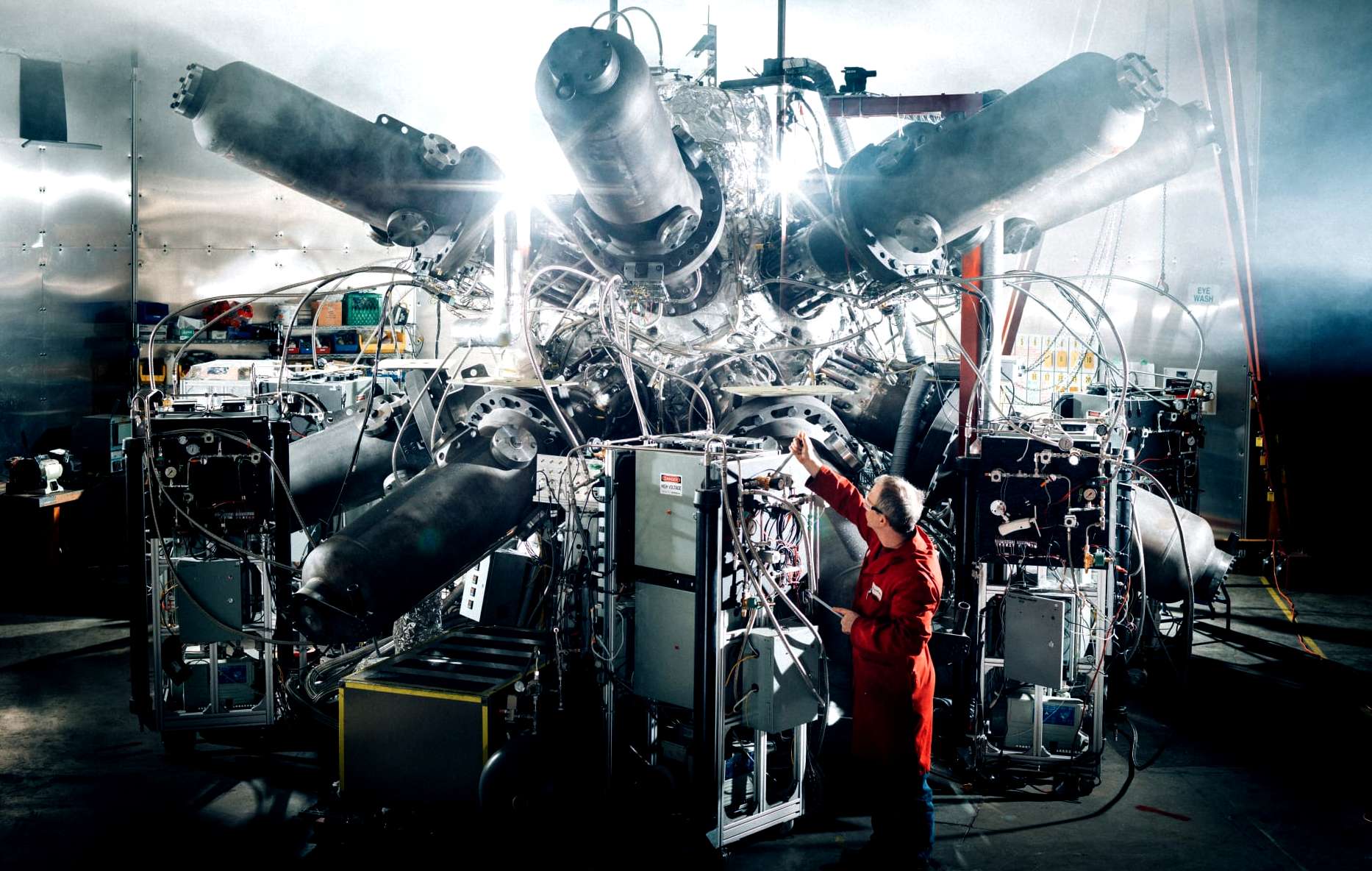
ACCIDENTS
Accidents in nuclear power plants include the Chernobyl disaster in the Soviet Union in 1986, the Fukushima Daiichi nuclear disaster in Japan in 2011, and the more contained Three Mile Island accident in the United States in 1979.
The first major nuclear accidents were the Kyshtym disaster in the Soviet Union and the Windscale fire in the United Kingdom, both in 1957. The first major accident at a nuclear reactor in the USA occurred in 1961 at the SL-1, a U.S. Army experimental nuclear power reactor at the Idaho National Laboratory. An uncontrolled chain reaction resulted in a steam explosion which killed the three crew members and caused a meltdown. Another serious accident happened in 1968, when one of the two liquid-metal-cooled reactors on board the Soviet submarine K-27 underwent a fuel element failure, with the emission of gaseous fission products into the surrounding air, resulting in 9 crew fatalities and 83 injuries.
Some serious nuclear and radiation accidents have occurred. The severity of nuclear accidents is generally classified using the International Nuclear Event Scale (INES) introduced by the International Atomic Energy Agency (IAEA). The scale ranks anomalous events or accidents on a scale from 0 (a deviation from normal operation that poses no safety risk) to 7 (a major accident with widespread effects). There have been 3 accidents of level 5 or higher in the civilian nuclear power industry, two of which, the Chernobyl accident and the Fukushima accident, are ranked at level 7.
The Fukushima Daiichi nuclear accident was caused by the 2011 Tohoku earthquake and tsunami. The accident has not caused any radiation-related deaths but resulted in radioactive contamination of surrounding areas. The difficult cleanup operation is expected to cost tens of billions of dollars over 40 or more years. The Three Mile Island accident in 1979 was a smaller scale accident, rated at INES level 5. There were no direct or indirect deaths caused by the accident.
[There is one recorded death from radiation poisoning]
The impact of nuclear accidents is controversial. According to Benjamin K. Sovacool, fission energy accidents ranked first among energy sources in terms of their total economic cost, accounting for 41 percent of all property damage attributed to energy accidents. Another analysis found that
coal, oil, liquid
petroleum gas and hydroelectric accidents (primarily due to the Banqiao Dam disaster) have resulted in greater economic impacts than nuclear power accidents. The study compares latent cancer deaths attributable to nuclear with immediate deaths from other energy sources per unit of energy generated, and does not include
fossil fuel related cancer and other indirect deaths created by the use of fossil fuel consumption in its "severe accident" (an accident with more than 5 fatalities) classification. The Chernobyl accident in 1986 caused approximately 50 deaths from direct and indirect effects, and some temporary serious injuries from acute radiation syndrome. The future predicted mortality from increases in cancer rates is estimated at about 4000 in the decades to come. However, the costs have been large and are increasing.
Extreme weather events, including events made more severe by climate change, decrease the reliability of nuclear energy. Novel reactor types and weakening of safety standards to increase competitiveness of nuclear energy may increase risks or have new risks of accidents.
Nuclear power works under an insurance framework that limits or structures accident liabilities in accordance with national and international conventions. It is often argued that this potential shortfall in liability represents an external cost not included in the cost of nuclear electricity. This cost is small, amounting to about 0.1% of the levelized cost of
electricity, according to a study by the Congressional Budget Office in the United States. These beyond-regular insurance costs for worst-case scenarios are not unique to nuclear power. The State effectively underwrites the remainder, having granted planning consent - so assumes a vicarious liability in terms of ecocide. 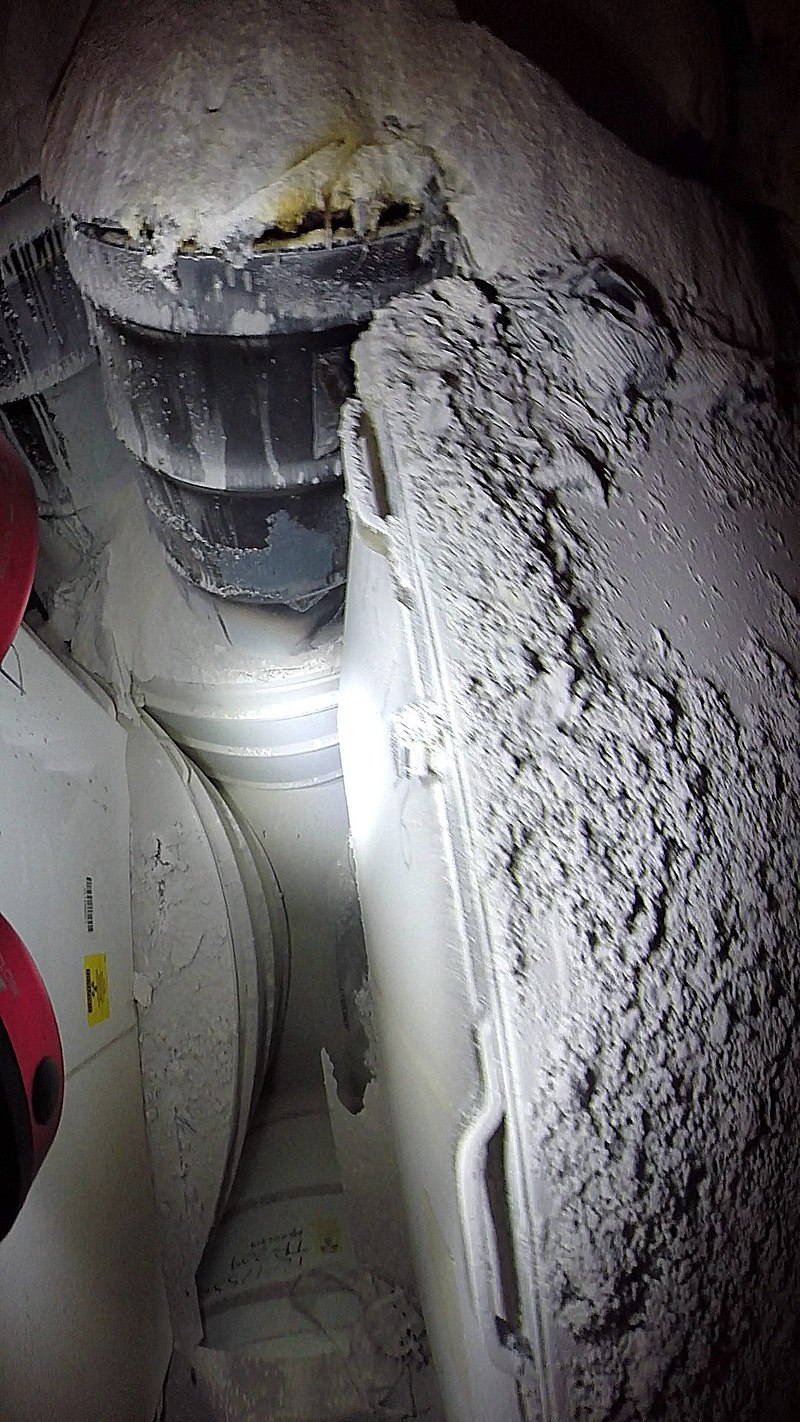
TERRORIST ATTACKS AND SABOTAGE
Terrorists could target nuclear power plants in an attempt to release radioactive contamination into the community. The United States 9/11 Commission has said that nuclear power plants were potential targets originally considered for the September 11, 2001 attacks. An attack on a reactor's spent fuel pool could also be serious, as these pools are less protected than the reactor core. The release of radioactivity could lead to thousands of near-term deaths and greater numbers of long-term fatalities.
In the United States, the NRC carries out "Force on Force" (FOF) exercises at all nuclear power plant sites at least once every three years. In the United States, plants are surrounded by a double row of tall fences which are electronically monitored. The plant grounds are patrolled by a sizeable force of armed guards.
Insider sabotage is also a threat because insiders can observe and work around security measures. Successful insider crimes depended on the perpetrators' observation and knowledge of security vulnerabilities. A fire caused 5–10 million dollars worth of damage to New York's Indian Point Energy Center in 1971. The arsonist turned out to be a plant maintenance worker.
NUCLEAR PROLIFERATION
Nuclear proliferation is the spread of nuclear weapons, fissionable material, and weapons-related nuclear technology to states that do not already possess nuclear weapons. Many technologies and materials associated with the creation of a nuclear power program have a dual-use capability, in that they can also be used to make nuclear weapons. For this reason, nuclear power presents proliferation risks.
Nuclear power program can become a route leading to a nuclear weapon. An example of this is the concern over Iran's nuclear program. The re-purposing of civilian nuclear industries for military purposes would be a breach of the Non-proliferation treaty, to which 190 countries adhere. As of April 2012, there are thirty one countries that have civil nuclear power plants, of which nine have nuclear weapons. The vast majority of these nuclear weapons states have produced weapons before commercial nuclear power stations.
A fundamental goal for global security is to minimize the nuclear proliferation risks associated with the expansion of nuclear power. The Global Nuclear Energy Partnership was an international effort to create a distribution network in which developing countries in need of energy would receive nuclear fuel at a discounted rate, in exchange for that nation agreeing to forgo their own indigenous development of a uranium enrichment program. The France-based Eurodif/European Gaseous Diffusion Uranium Enrichment Consortium is a program that successfully implemented this concept, with Spain and other countries without enrichment facilities buying a share of the fuel produced at the French-controlled enrichment facility, but without a transfer of technology. Iran was an early participant from 1974 and remains a shareholder of Eurodif via Sofidif.
A 2009 United Nations report said that:
" ..the revival of interest in nuclear power could result in the worldwide dissemination of uranium enrichment and spent fuel reprocessing technologies, which present obvious risks of proliferation as these technologies can produce fissile materials that are directly usable in nuclear weapons."
On the other hand, power reactors can also reduce nuclear weapons arsenals when military-grade nuclear materials are reprocessed to be used as fuel in nuclear power plants. The Megatons to Megawatts Program is considered the single most successful non-proliferation program to date. Up to 2005, the program had processed $8 billion of high enriched, weapons-grade uranium into low enriched
uranium suitable as nuclear fuel for commercial fission reactors by diluting it with natural uranium. This corresponds to the elimination of 10,000 nuclear weapons. For approximately two decades, this material generated nearly 10 percent of all the electricity consumed in the United States, or about half of all U.S. nuclear electricity, with a total of around 7,000 TWh of electricity produced. MEGATONS
TO MEGAWATTS In total it is estimated to have cost $17 billion, a "bargain for US ratepayers", with Russia profiting $12 billion from the deal. Much needed profit for the Russian nuclear oversight industry, which after the collapse of the Soviet economy, had difficulties paying for the maintenance and security of the Russian Federations highly enriched uranium and warheads. The Megatons to Megawatts Program was hailed as a major success by anti-nuclear weapon advocates as it has largely been the driving force behind the sharp reduction in the number of nuclear weapons worldwide since the cold war ended. However, without an increase in nuclear reactors and greater demand for fissile fuel, the cost of dismantling and down blending has dissuaded Russia from continuing their disarmament. As of 2013 Russia appears to not be interested in extending the program. It
is unsustainable thinking to allow Russia to believe that their nuclear
missile programme and retirement of dangerous waste might be subsidised by
US, UK, EU, or any other country's thirst for cheap electricity.
It should never be that military swank is underpinned by irradiating
citizens, and continuing to dump the un-dump-able. What is needed is a Radiation
Tax, to reflect the true cost of managing future accidents and
responsibly managed waste disposal for thousands of years to come. 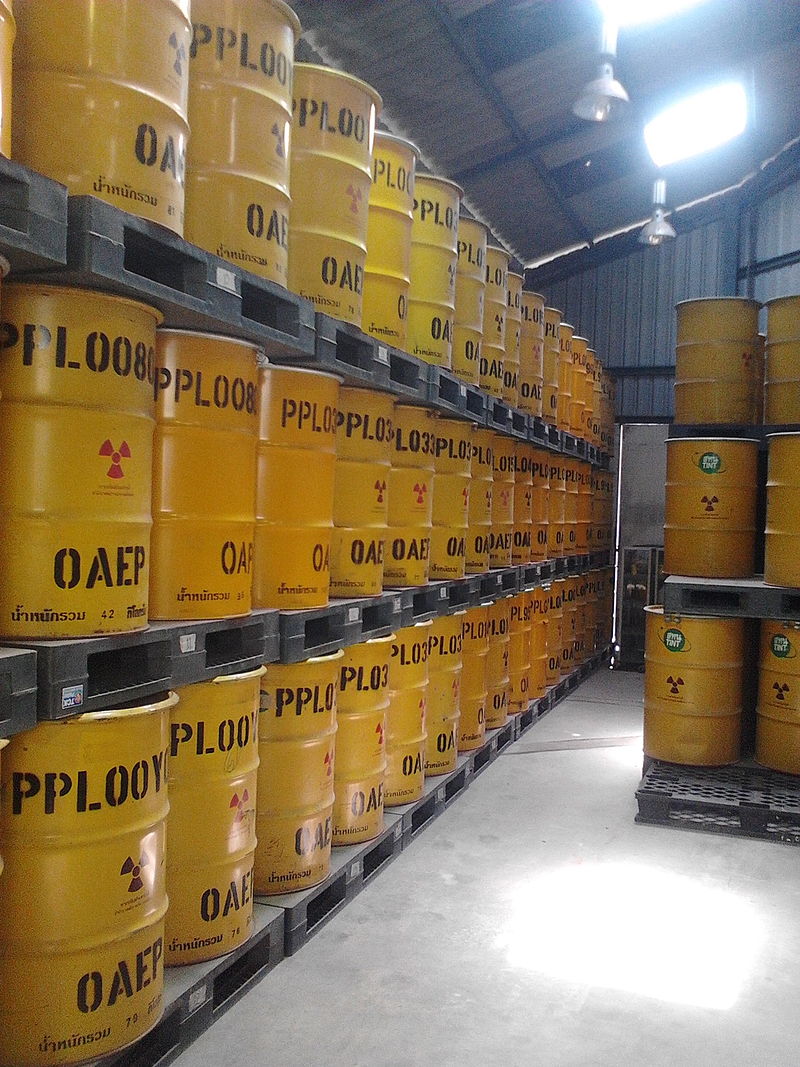
WASTE DISPOSAL AND STORAGE
Following interim storage in a spent fuel pool, the bundles of used fuel rod assemblies of a typical nuclear power station are often stored on site in dry cask storage vessels. Presently, waste is mainly stored at individual reactor sites and there are over 430 locations around the world where radioactive material continues to accumulate.
Disposal of nuclear waste is often considered the most politically divisive aspect in the lifecycle of a nuclear power facility. With the lack of movement of nuclear waste in the 2 billion year old natural nuclear fission reactors in Oklo, Gabon being cited as "a source of essential information today." Experts suggest that centralized underground repositories which are well-managed, guarded, and monitored, would be a vast improvement. There is an "international consensus on the advisability of storing nuclear waste in deep geological repositories". With the advent of new technologies, other methods including horizontal drillhole disposal into geologically inactive areas have been proposed.
Most waste packaging, small-scale experimental fuel recycling chemistry and radiopharmaceutical refinement is conducted within remote-handled hot cells.
There are no commercial scale purpose built underground high-level waste repositories in operation. However, in Finland the Onkalo spent nuclear fuel repository of the Olkiluoto Nuclear Power Plant is under construction as of 2015.
The most important waste stream from nuclear power reactors is spent nuclear fuel, which is considered high-level waste. For LWRs, spent fuel is typically composed of 95% uranium, 4% fission products, and about 1% transuranic actinides (mostly plutonium, neptunium and
americium). The plutonium and other transuranics are responsible for the bulk of the long-term radioactivity, whereas the fission products are responsible for the bulk of the short-term radioactivity.
High-level waste requires treatment, management, and isolation from the environment. These operations present considerable challenges due to the extremely long periods these materials remain potentially hazardous to living organisms. This is due to long-lived fission products (LLFP), such as technetium-99 (half-life 220,000 years) and iodine-129 (half-life 15.7 million years). LLFP dominate the waste stream in terms of radioactivity, after the more intensely radioactive short-lived fission products (SLFPs) have decayed into stable elements, which takes approximately 300 years. Due to the exponential decrease of radioactivity with time, spent nuclear fuel activity decrease by 99.5% after 100 years. After about 100,000 years, the spent fuel becomes less radioactive than natural uranium ore. Commonly suggested methods to isolate LLFP waste from the biosphere include separation and transmutation, synroc treatments, or deep geological storage.
Thermal-neutron reactors, which presently constitute the majority of the world fleet, cannot burn up the reactor grade plutonium that is generated during the reactor operation. This limits the life of nuclear fuel to a few years. In some countries, such as the United States, spent fuel is classified in its entirety as a nuclear waste. In other countries, such as France, it is largely reprocessed to produce a partially recycled fuel, known as mixed oxide fuel or MOX. For spent fuel that does not undergo reprocessing, the most concerning isotopes are the medium-lived transuranic elements, which are led by reactor-grade plutonium (half-life 24,000 years). Some proposed reactor designs, such as the Integral Fast Reactor and molten salt reactors, can use as fuel the plutonium and other actinides in spent fuel from light water reactors, thanks to their fast fission spectrum. This offers a potentially more attractive alternative to deep geological disposal.
The thorium fuel cycle results in similar fission products, though creates a much smaller proportion of transuranic elements from neutron capture events within a reactor. Spent thorium fuel, although more difficult to handle than spent uranium fuel, may present somewhat lower proliferation risks.
DEEP GEOLOGICAL DEPOSITORIES
A deep geological repository is a way of storing hazardous or radioactive waste within a stable geologic environment (typically 200–1000 m deep). It entails a combination of waste form, waste package, engineered seals and geology that is suited to provide a high level of long-term isolation and containment without future maintenance. This will prevent any radioactive dangers. A number of mercury, cyanide and arsenic waste repositories are operating worldwide including Canada (Giant Mine) and Germany (potash mines in Herfa-Neurode and Zielitz) and a number of radioactive waste storages are under construction with the Onkalo in Finland being the most advanced.
OCEAN FLOOR DISPOSAL
Ocean floor disposal is a method of sequestering radioactive waste in ocean floor sediment where it is unlikely to be disturbed either geologically or by human activity.
Several methods of depositing material in the ocean floor have been proposed, including encasing it in concrete and as the United Kingdom has previously done, dropping it in torpedoes designed to increase the depth of penetration into the ocean floor, or depositing containers in shafts drilled with techniques similar to those used in oil
exploration.
Ocean floor sediment is saturated with water, but since there is no water table per se and the water does not flow through it the migration of dissolved waste is limited to the rate at which it can diffuse through dense clay. This is slow enough that it could potentially take millions of years for waste to diffuse through several tens of meters of sediment so that by the time it reaches open ocean it would be highly dilute and decayed. Large regions of the ocean floor are thought to be completely geologically inactive and it is not expected that there will be extensive human activity there in the future. Water absorbs essentially all radiation within a few meters provided the waste remains contained.
One of the problems associated with this option includes the difficulty of recovering the waste, if necessary, once it is emplaced deep in the ocean. Also, establishing an effective international structure to develop, regulate, and monitor a sub-seabed repository would be extremely difficult.
Beyond technical and political considerations, the London Convention places prohibitions on disposing of radioactive materials at sea and does not make a distinction between waste dumped directly into the water and waste that is buried underneath the ocean's floor. It remained in force until 2018, after which the sub-seabed disposal option can be revisited at 25-year intervals.
Depositing waste, in suitable containers, in subduction zones has also been suggested. Here, waste would be transported by plate tectonic movement into the Earth's mantle and rendered harmless through dilution and natural decay. Several objections have been raised to this method, including vulnerabilities during transport and disposal, as well as uncertainties in the actual tectonic processes.
DEEP BOREHOLE DISPOSAL
Deep borehole disposal (DBD) is the concept of disposing high-level radioactive waste from nuclear reactors in extremely deep boreholes instead of in more traditional deep geological repositories that are excavated like mines. Deep borehole disposal seeks to place the waste as much as five kilometres (3.1 mi) beneath the surface of the Earth and relies primarily on the thickness of the natural geological barrier to safely isolate the waste from the biosphere for a very long period of time so that it should not pose a threat to humans and the environment. The concept was originally developed in the 1970s, but in 2014 a proposal for a first experimental borehole was proposed by a consortium headed by Sandia National Laboratories.
The waste would be put into the lower mile of such a hole, within crystalline rock to isolate it from the environment. The upper two miles of the borehole would be filled with protective layers including asphalt, bentonite, concrete and crushed rock that are expected to protect the environment during geologic time, and the hole would be lined with steel casing.
A pair of proposed test boreholes in the United States were cancelled due to public opposition and lack of funding in 2016 and 2017.
Beginning in 2016, the U.S. Department of Energy funded an experimental borehole extending over 3 miles (4.8 km) deep, in Rugby, North Dakota. The plans for this five-year project in Rugby did not involve nuclear waste, and instead would have tested other aspects of the borehole concept. However, following protests in North Dakota, a site was proposed in Spink County, South Dakota. After protests in South Dakota prevented the project from moving forward, the Department of Energy scrapped the project. Due to public opposition to the first experimental borehole, in late 2016 the Department of Energy announced a second project which would have involved four sites; two in New Mexico, one in Texas and one in South Dakota. The early stages of the project required gaining public support before the Department of Energy would have selected a final site for an experimental borehole. On 23 May 2017, the Department of Energy announced that funding priorities had changed and that the deep borehole project was de-funded.
MANAGEMENT
Of particular concern in nuclear waste management are two long-lived fission products, Tc-99 (half-life 220,000 years) and I-129 (half-life 15.7 million years), which dominate spent fuel radioactivity after a few thousand years. The most troublesome transuranic elements in spent fuel are Np-237 (half-life two million years) and Pu-239 (half-life 24,000 years). Nuclear waste requires sophisticated treatment and management to successfully isolate it from interacting with the biosphere. This usually necessitates treatment, followed by a long-term management strategy involving storage, disposal or transformation of the waste into a non-toxic form. Governments around the world are considering a range of waste management and disposal options, though there has been limited progress toward long-term waste management solutions.
In the second half of the 20th century, several methods of disposal of radioactive waste were investigated by nuclear nations, which are :
- "Long-term above-ground storage", not implemented. -
"Disposal in outer space" (for instance, inside the Sun), not implemented -
as it would be currently too expensive. -
"Deep borehole disposal", not implemented. -
"Rock melting", not implemented. -
"Disposal at subduction zones", not implemented. -
Ocean disposal, by the USSR, the United Kingdom, Switzerland, the United States, Belgium, France, the Netherlands, Japan, Sweden, Russia, Germany, Italy and South Korea (1954–93). This is no longer permitted by international agreements. -
"Sub-seabed disposal", not implemented, not permitted by international agreements. -
"Disposal in ice sheets", rejected in Antarctic Treaty -
"Direct injection", by USSR and USA. -
Nuclear transmutation, using lasers to cause beta decay to convert the unstable atoms to those with shorter half-lives.
In the United States, waste management policy completely broke down with the ending of work on the incomplete Yucca Mountain Repository. At present there are 70 nuclear power plant sites where spent fuel is stored. A Blue Ribbon Commission was appointed by
President Obama to look into future options for this and future waste. A deep geological repository seems to be favored. 2018 Nobel Prize for Physics-winner Gérard Mourou has proposed using Chirped pulse amplification to generate high-energy and low-duration laser pulses to transmute highly radioactive material (contained in a target) to significantly reduce its half-life, from thousands of years to only a few minutes.
In January 2013, Cumbria County Council rejected UK central government proposals to start work on an underground storage dump for nuclear waste near to the Lake District National Park.
"For any host community, there will be a substantial community benefits package and worth hundreds of millions of pounds" said Ed Davey,
Energy
Secretary, but nonetheless, the local elected body voted 7–3 against research continuing, after hearing evidence from independent geologists that "the fractured strata of the county was impossible to entrust with such dangerous material and a hazard lasting millennia."
ECONOMICS
The levelized cost of electricity (LCOE) from a new nuclear power plant is estimated to be 69 USD/MWh, according to an analysis by the International Energy Agency and the OECD Nuclear Energy Agency. This represents the median cost estimate for an nth-of-a-kind nuclear power plant to be completed in 2025, at a discount rate of 7%. Nuclear power was found to be the least-cost option among dispatchable technologies. Variable renewables can generate cheaper electricity: the median cost of onshore wind power was estimated to be 50 USD/MWh, and utility-scale solar power 56 USD/MWh. At the assumed CO2 emission cost of USD 30 per ton, power from coal (88 USD/MWh) and gas (71 USD/MWh) is more expensive than low-carbon technologies.
With
renewables being some $13 - 19 dollars cheaper per MWh, it's a no-brainer
that nuclear power should be a non-starter. Why invest in a bum
deal, potentially up
to your neck in legal sewage. Consider also, that there is as yet no
absolutely sure way to dispose of radioactive waste, with half lives of
hundreds of thousands of years. No management plan in the world is going to
survive a few generations of humans, after which the corporations or other
organizations will cease to exist or care - and conveniently wash their
hands of the liability. If
ever this was a case of ecocide for the International Criminal Court, we
don't know what is. At the moment, almost any waste management plan
forensically examined, will prove to be defective, negligent, and so
potentially harmful to planet earth. Some
investors may not care about that, for they will be dead and buried, but for
the sake of their great
grandchildren, they might want to consider investing in renewables.
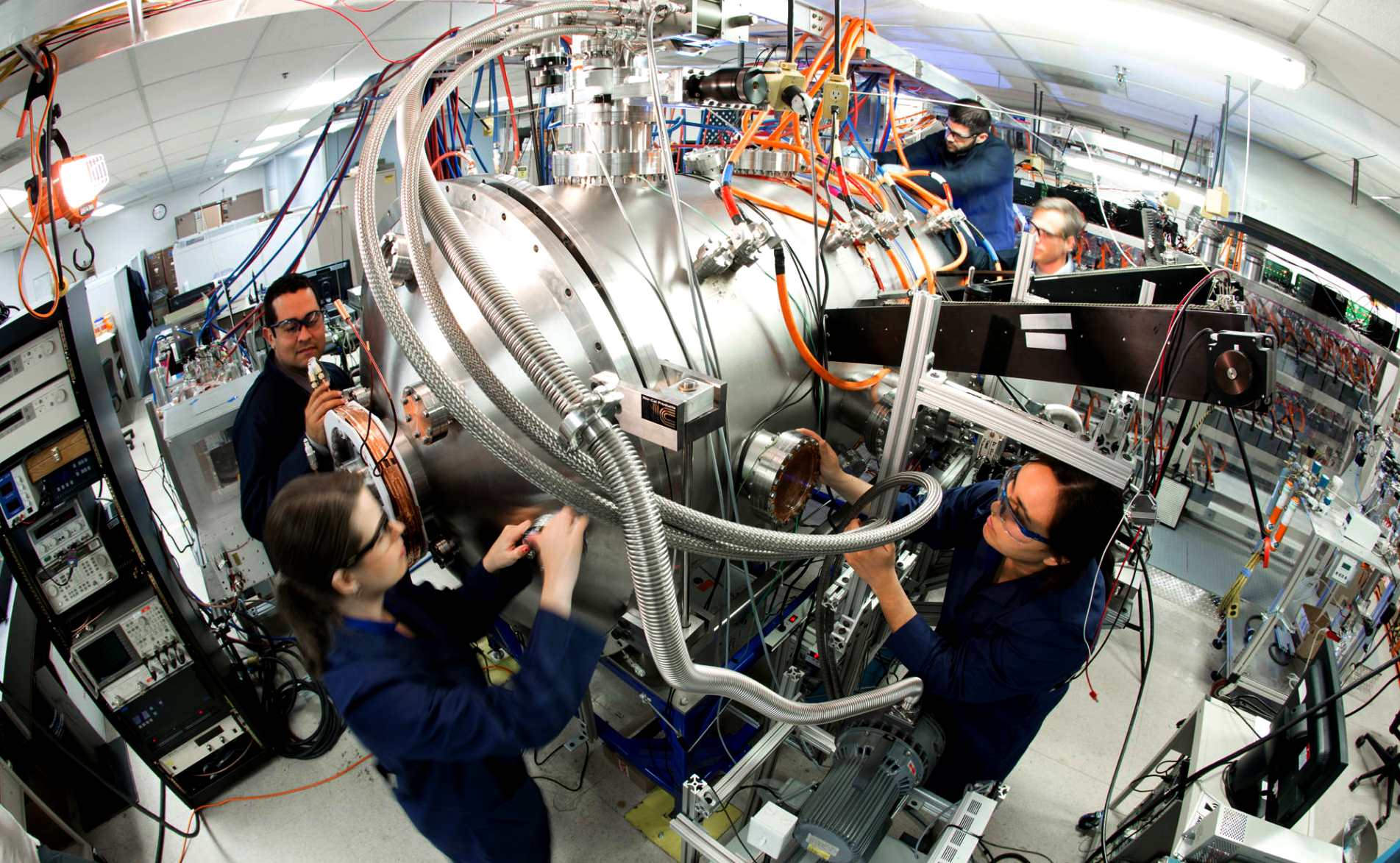
As U.S. Moves Ahead with Nuclear Power, No Solution for Radioactive Waste Experts Divided Over Safety of Indian Point Nuclear Power Plant Japanese Disaster May Affect India’s Import of Foreign Nuclear Reactors.
LONG TERM
INSECURITY
In
the short term we are reliant
on fossil fuels to take us into a
sustainable age where a circular economy is recognized as essential to
harmonious living. Long-term measures to increase energy security center on reducing dependence on any one source of imported energy, increasing the number of suppliers, exploiting native
fossil fuel or renewable energy resources, and reducing overall demand through energy conservation measures.
We
might also enter into international agreements to undermine fossil fuel energy trading
monopolies and assure that everyone has the right to cheap and clean
renewable energy, with the need to transport imports. Those
who held the power and wealth should consider re-investing in alternatives
as they head towards the sustainable economics of zero growth.
The deployment of renewable
energy technologies increases the diversity of electricity sources and contributes to the flexibility of
an international infrastructure system and its resistance to central shocks,
especially where off-grid installations are widely deployed, but can be grid
connected.
It
is likely to be that at some point in the future we will no longer need
power stations that run on coal, oil
or nuclear fuels. We will have dragged ourselves out of the fossil
fuel cesspit and taken power generation from the fortunate few who
profit from geological deposits, to the masses who only need a space to
mount the harvesting medium for energy independence.
For those countries
whose reliance on imported
gas is a significant
energy security issue, renewable technologies can provide
a level playing field.
As the fossil resources that have been so crucial to human advancement start declining in numbers, countries will
be glad that they changed over to renewable energy. When it comes to decommissioning
a nuclear power plant, or when waste material escapes into the environment,
the nuclear lobby may want to think again.
FUSION | BIOFUELS
| GEOTHERMAL
| HYDRO-ELECTRIC
| SOLAR |
WAVE
& TIDAL | WIND
COMPRESSED
GAS - ECONOMY - FUEL
CELLS - FUSION
- HYDRIDES
- LIQUID GAS
FUSION
LINKS
& REFERENCE
Plutonium
https://www.
Please
use our A-Z
INDEX to navigate this site
|